How Does the Signal Travel?
Episode #4 of the course Understanding your brain by Betsy Herbert
Welcome back!
Last lesson, we learned about the tiny electrical events known as action potentials, which occur along the neuron. This lesson, we’ll learn how a string of these action potentials fire in succession to generate a signal that “travels” along the neuron.
Saltatory Conduction
The first thing to note is that once an action potential has fired in one particular section of axon, that section becomes inactive, while ion exchangers get to work restoring the careful balance of the resting potential. We say that this section is in its refractory period. It means that the only place an action potential can be triggered next is in the section of axon downstream of the signal, because all the sections upstream are still recovering.
This creates a very important fact about the neuron’s signals: They are unidirectional, meaning they can only travel from dendrites to the axon and not the other way around. If they didn’t, our information wouldn’t get anywhere!
The second thing to note is that if action potentials were just triggered in adjacent sections of the axon very close to one another, the signal would take a long time to travel and we wouldn’t think very fast. So, our neurons have evolved a very clever mechanism to hurry the signal along: They’re coated in an insulating sheath of fatty tissue known as a myelin sheath. Small gaps in this sheath, called Nodes of Ranvier, occur at periodic points along the axon. Because ions cannot pass across the insulating layer, the only points where action potentials can be triggered are at precisely these nodes, so they effectively “jump” from node to node, increasing the speed of signal propagation. This process is known as saltatory conduction.
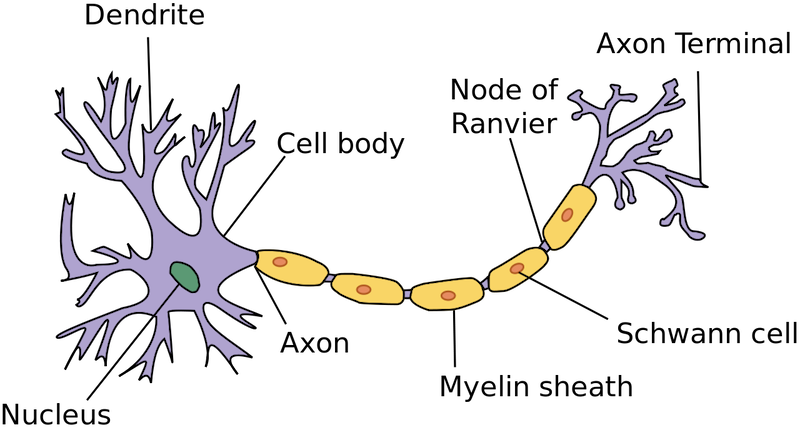
Saltatory conduction occurs only on myelinated axons.
So, now we have a signal that can pass very quickly along the neuron, right to the tip of its axon. But what happens when it gets there? How does the signal pass to the next neuron?
Synaptic Transmission
Where one neuron’s axon meets the next neuron’s dendrite, there’s a tiny gap—just 20 nm wide—known as a synapse. Electricity cannot jump across this gap, so the signal is briefly carried instead by chemicals. Tiny molecules called neurotransmitters stored at the tip of the axon are released when an action potential arrives, diffusing across the gap and binding to specialized receptors on the other side.
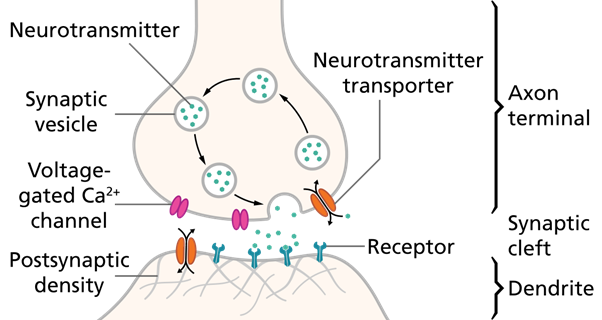
The presynaptic neuron (top) releases neurotransmitters, which activates receptors on the postsynaptic cell (bottom).
Depending on the neurotransmitter, this can either help the next neuron generate a new action potential or make it much harder for it to do so. Neurotransmitters are therefore classed into two broad categories: “excitatory” or “inhibitory.”
It’s strange to think that inhibiting a signal can sometimes be helpful, but in fact, it’s an essential part of information processing. After all, the signal mustn’t spread out in every direction around the brain, activating everything all at once—it needs to go in select paths. Here are examples of common neurotransmitters. Have you heard of any?
• excitatory: glutamate, acetylcholine, adrenaline
• inhibitory: GABA (gamma-aminobutyric acid), glycine
• both: dopamine, serotonin, noradrenaline
It’s important to note that the categories are not clear cut, and most neurotransmitters can be both excitatory and inhibitory depending on their location and receptors. Some neurotransmitters, such as glutamate, are found all over the brain and carry almost every type of signal, while others are found in specific areas and have more isolated roles. For example, you may have heard that some neurotransmitters, such as dopamine and serotonin, have a role in regulating our mood. Others have a key role in voluntary behavior, sleep, memory, and reward signals. We’ll explore all this exciting “brain chemistry” in later lessons.
But first: How does information enter the brain in the first place? In the next few lessons, we’ll be taking a tour of the senses and understanding how signals from the outside world fill our brain with life.
See you tomorrow!
Betsy
Recommended video
The Nervous System, Part 3 — Synapses!
Recommended reading
Neurotransmitters and Receptors
Share with friends